A Potential Breakthrough in Astrophysics: Decoding the Universe Through Pulsar Timing Arrays
The world of astrophysics is buzzing with excitement ahead of an announcement by the International Pulsar Timing Array (IPTA) this Thursday.
Rumours are flying around that the announcement could revolutionise our understanding of the universe, physics, and time. We will have to wait another few days to see if it lives up to the hype.
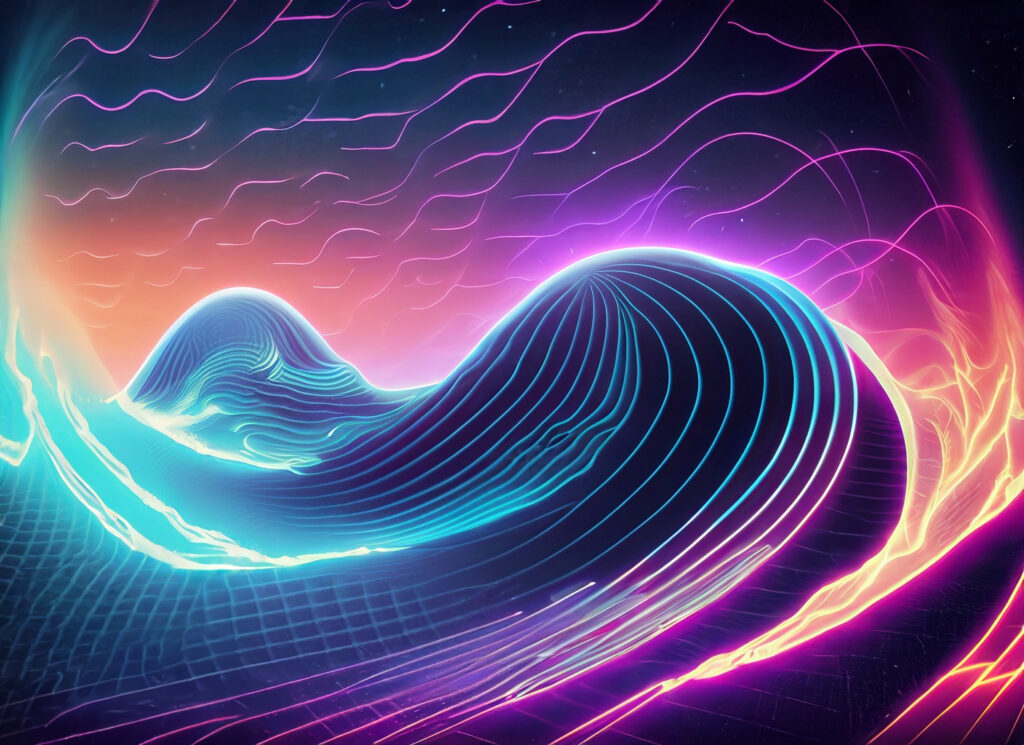
Whatever the announcement, the International Pulsar Timing Array (IPTA) is a fascinating blending of scientific innovation and engineering expertise. Let us get up to speed on the International Pulsar Timing Array (IPTA) and how we can use Pulsar Timings to understand the early universe.
The International Pulsar Timing Array (IPTA) is a global consortium that includes gravitational wave detectors like North America’s NANOGrav, the European Pulsar Timing Array, the Indian Pulsar Timing Array Project, and Australia’s Parkes Pulsar Timing Array.
Pulsar Timing Arrays: Harnessing the Power of Cosmic Lighthouses
In simple terms, the buzz comes from a method of gravitational wave detection that uses ‘pulsar timing arrays’.
These arrays rely on the consistent emissions of pulsars, neutron stars that spin at phenomenal speeds, to detect the subtle shifts caused by the passage of gravitational waves.
Until now, our knowledge of gravitational waves comes from laser interferometers like LIGO and Virgo. These instruments are excellent at capturing high-frequency gravitational waves. Pulsar Timing Arrays, however, offer us an alternative viewpoint—providing us with a window into low-frequency gravitational waves. The detection and study of these low-frequency waves could unveil secrets about the formation and evolution of supermassive black hole binaries, galaxy formation, and the early universe.
Think of the still pond as the fabric of spacetime. The ripple created by throwing a stone in it is akin to a gravitational wave emitted from a massive cosmic event, such as merging black holes or neutron stars.
We detect these ripples, or gravitational waves, using something like a giant ruler, but not just any ruler which can measure space shortening; we use a specific type of ruler known as a laser interferometer. This “ruler” aims to measure the minute distortions in spacetime caused by the passing of a gravitational wave.
This laser interferometer splits a laser beam into two halves, sending each along two different paths at a right angle to each other. In a scenario where no gravitational waves pass through, these two beams of light travel the same distance and recombine to produce a stable pattern.
However, when a gravitational wave — our spacetime ripple — passes through, it temporarily distorts spacetime. This distortion is akin to stretching one part of your ruler and squishing another. The result is that one beam of light now has a slightly longer path to travel and the other a slightly shorter one, similar to how a ripple would make one part of a pond momentarily deeper and another shallower.
When these light beams recombine, they are no longer in perfect sync due to their differing path lengths. This causes a shift in the interference pattern, like marks on a ruler moving, and this is what the detector picks up.
Despite these changes being incredibly small (smaller than one ten-thousandth the diameter of a proton), these “rulers” or laser interferometers are sensitive enough to detect them. By recording these changes, we can detect the presence of gravitational waves, essentially ‘seeing’ the ripples in our spacetime pond caused by the most influential events in the universe.
When we are talking about measuring gravitational waves, the scale of the measurement apparatus is crucial. The primary reason is that the effect of gravitational waves is proportional to the distance over which the measurement is made. Essentially, the larger the “ruler” (or, in this case, the interferometer), the more significant the observed effect of a passing gravitational wave.
So, if we could measure over several light years, the tiny distortions in spacetime caused by gravitational waves would accumulate over that vast distance and become much easier to detect. In other words, the stretching and squeezing effects of the wave would be magnified, making them more readily detectable.
Building a detector at this scale is impossible. However, scientists have long theorised that we could use pulsars as ready-made detectors. Imagine pulsars as cosmic lighthouses meticulously distributed across the galaxy.
They emit beams of radio waves with extraordinary regularity. By closely monitoring this network of pulsars, astronomers can accurately measure the arrival of pulses from these distant celestial bodies.
This timing technique has the sensitivity to detect minuscule shifts, providing us with vital clues about the influence of gravitational waves.
What Are Gravitational Waves: Einstein’s Legacy
Proposed by Einstein’s theory of general relativity, gravitational waves are distortions in the fabric of spacetime triggered by massive astrophysical events.
These waves propagate across the universe at the speed of light, causing the pulsar signals’ arrival times on Earth to shift. We gain insight into the colossal cosmic events that created these waves through their detection.
The beauty of using pulsar timing arrays is their remarkable sensitivity. They can theoretically detect gravitational waves originating from supermassive black hole binaries—pairs of black holes, each million to billions of times the mass of our Sun. These celestial pairs are engaged in an intricate cosmic dance stretching for billions of years.
Gravitational wave astronomy: Unveiling the Universe’s Secrets with PTAs
Up to now, this has all been theoretical. The announcement is believed that PTAs may have detected these elusive low-frequency gravitational waves for the first time. If true, this would signify a significant leap forward in gravitational wave astronomy and significantly expand our understanding of the universe.
The implications of this discovery extend far beyond the realm of astronomy. It could offer a novel approach to test the limitations of Einstein’s theory of relativity and deepen our understanding of the fundamental laws of physics.
Gravitational wave astronomy opens a new way of understanding and exploring the universe. Traditional astronomy relies on electromagnetic radiation – like light, radio waves, and x-rays.- all forms of light that various telescopes can detect. However, gravitational waves offer a different way of gathering information about cosmic events, including:
- Observing Invisible Phenomena: Gravitational waves allow us to observe events that do not emit light or other electromagnetic radiation or are obscured in some way. This includes things like the merging of black holes or neutron stars.
- Testing General Relativity: Gravitational waves give us a new way to test Einstein’s theory of general relativity in extreme conditions, such as the powerful gravitational fields found near black holes.
- Understanding the Early Universe: Gravitational waves could allow us to study the early universe in ways electromagnetic radiation cannot. The Cosmic Microwave Background (CMB) radiation gives us a picture of the universe about 380,000 years after the Big Bang, but before that, the universe was too hot and dense for light to travel freely. On the other hand, Gravitational waves could give us a glimpse of the universe just fractions of a second after the Big Bang.
- Detecting Unknown Phenomena: We can always detect entirely new phenomena that we have yet to predict.
- Neutron Star Insights: By studying the gravitational waves produced by the collisions of neutron stars, scientists could learn more about their internal structure, which is still a mystery.
- Dark Matter and Dark Energy: While it is still speculative, gravitational waves may help us better understand the nature of dark matter and dark energy.
Gravitational wave astronomy adds another critical tool to the astronomer’s toolbox, giving us a whole new way to look at and understand the universe.
How do Pulsar Timing Arrays work?
Pulsar Timing Arrays (PTAs) serve as our unique lens into the universe. They allow us to “listen” to the cosmic symphony and unravel some of the most profound mysteries of the cosmos. The rapid, consistent rotation of pulsars and their emitted beams of electromagnetic radiation makes them particularly useful for PTAs.
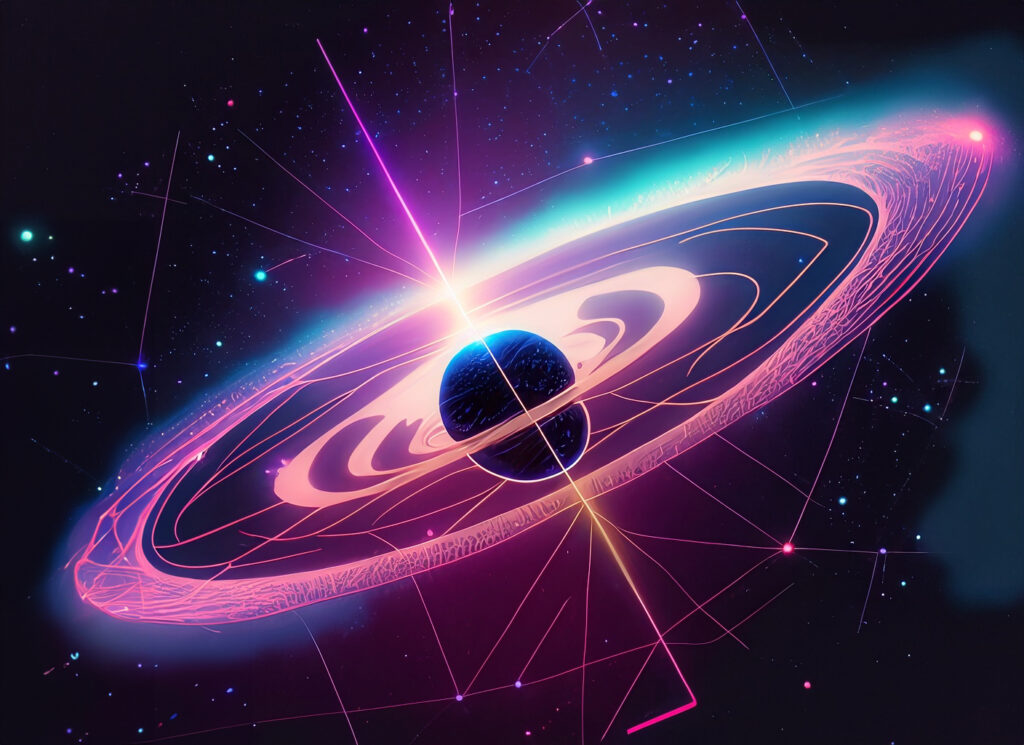
Pulsar timing involves meticulously measuring the times of arrival (TOAs) of pulses emitted by pulsars. Any deviation in these TOAs can indicate changes in the medium through which the signals pass, including spacetime distortions caused by gravitational waves.
Radio telescopes capture these signals, and the data undergoes extensive analysis to create a comprehensive “timing model” for each pulsar.
This model considers various factors, such as the pulsar’s spin period, spin-down rate, and location.
Computer algorithms then compare the observed TOAs with the predicted TOAs from the timing model.
If gravitational waves have traversed the space between the pulsar and Earth, they will cause a correlated change in the TOAs of a group of pulsars—this correlation is what PTAs diligently seek.
Who is part of the International Pulsar Timing Array (IPTA)
The International Pulsar Timing Array (IPTA) is a testament to global scientific collaboration. By sharing data among regional PTAs, including NANOGrav, the European Pulsar Timing Array, and the Parkes Pulsar Timing Array, the IPTA creates a more accurate and sensitive timing array.
Engineering PTAs is a challenging task. It requires maintaining the precision of pulsar observations, dealing with random timing irregularities known as “red noise,” and continuously improving the sensitivity of radio telescopes. Advances in technology and computation play a pivotal role in overcoming these obstacles.
Pulsar Timing Arrays are a marvellous example of the harmonious integration of astrophysics, engineering, and data science. They enable us to “listen” to the cosmic symphony through an intricate interplay between scientific innovation and technical expertise.
We are eagerly anticipating the announcement on Thursday. We will write up the results for you, so follow us on Twitter, Linkedin, Instagram or Facebook for a breakdown of the potential discovery that could mark a new era in our quest to unravel the mysteries of the universe.
TL;DR:
- The International Pulsar Timing Array (IPTA) is rumoured to make an announcement that may revolutionise our understanding of the universe.
- Pulsar Timing Arrays (PTAs) use pulsars’ regular emissions to detect gravitational waves, offering a new perspective on low-frequency waves.
- PTAs can reveal information about supermassive black hole binaries, galaxy formation, and the early universe.
- Pulsars serve as cosmic lighthouses allowing us to measure the arrival times of pulses from these distant celestial bodies, detecting minuscule shifts caused by gravitational waves.
- Detecting gravitational waves allows us to observe invisible phenomena, test general relativity, understand the early universe, detect unknown phenomena, and gain insight into neutron stars, dark matter, and dark energy.
- The upcoming announcement from IPTA could signify a significant leap in gravitational wave astronomy and expand our understanding of the universe.