A nearly 200-year-old theoretical engine may be crucial in pursuing a sustainable, net zero future.
Carnot heat engines, first conceived in 1824 by French engineer Sadi Carnot, are the most efficient heat engines allowed by the laws of thermodynamics.
While practical limitations have historically prevented them, recent advances by a UK startup, Carnot Engines, could bring this technology into the 21st century, promising breakthroughs in efficiency and emissions.
The Carnot heat engine was born out of Sadi Carnot’s pioneering work exploring the efficiency of steam engines. In his 1824 treatise “Reflections on the Motive Power of Fire,” Carnot laid out a theoretical model for an engine operating between two heat reservoirs.
Using an idealized cycle of isothermal and adiabatic expansions and compressions, he showed that this engine’s efficiency depends solely on the reservoirs’ temperatures—not on the working fluid or engine details. In simpler terms, he proved that the maximum efficiency of a heat engine only depends on how hot and cold its heat sources are—it doesn’t matter what substance (like steam or gas) the engine uses or how the engine is built.
Over the following decades, eminent scientists like Émile Clapeyron and Rudolf Clausius developed Carnot’s ideas, making critical thermodynamic discoveries like entropy. The Carnot engine thus provided the framework for the emergence of thermodynamics as a scientific discipline. However, its seeming simplicity belied the immense practical challenges of building one.
Engineering Principles and Theory
We must dive into key thermodynamic concepts to understand how Carnot engines work.
At its core, a heat engine converts thermal energy into mechanical work. It operates between two heat reservoirs – a hot reservoir at temperature Th and a cold reservoir at Tc.
In each cycle, some heat Qh flows from the hot reservoir to the engine, the engine performs work W, and the remaining heat Qc is expelled to the cold reservoir.
The Carnot cycle that underpins the theoretical Carnot engine consists of four reversible processes:
- Isothermal expansion: The gas expands at constant temperature Th, absorbing heat Qh from the hot reservoir.
- Adiabatic expansion: The gas continues to expand without exchanging heat, working on the surroundings and cooling to Tc.
- Isothermal compression: The surroundings compress the gas at constant temperature Tc, expelling heat Qc to the cold reservoir.
- Adiabatic compression: The gas is compressed without heat transfer, raising its temperature to Th.
Carnot’s key insight was that this theoretical cycle represents the upper-efficiency limit for any heat engine operating between the same reservoirs.
The Carnot efficiency, a function only of the reservoir temperatures, is given by:
ηCarnot = 1 – (Tc/Th)
Where the temperatures are expressed on an absolute scale (Kelvin), crucially, reaching this efficiency requires the cycle to be fully reversible – meaning every process is quasi-static (gradual enough to maintain equilibrium), with no friction losses and heat exchange occurs only at the reservoir temperatures.
These requirements are impossible to achieve. Real engines face heat losses, friction, and imperfect heat exchange that permanently limit Carnot’s efficiency. Materials limits also constrain the maximum temperature differential. Nonetheless, the Carnot cycle is invaluable for understanding and improving real heat engines.
Engineers use the Carnot efficiency to gauge the performance of practical engine designs. For example, a modern diesel engine operates at around 42% efficiency. For its operating temperatures (Th ≈ 1000 K, Tc ≈ 400 K), the Carnot efficiency is roughly:
ηCarnot ≈ 1 – (400/1000) = 0.6 = 60%
Comparing the two values, the diesel engine achieves about 70% of the theoretical maximum efficiency. This gap represents the inescapable losses present in real-world systems.
Still, using high compression ratios, carefully timed fuel injections, and turbocharging to raise Th, diesel engines approach Carnot efficiency closer than most practical designs.
Other technologies use different strategies for nearing that ideal within their practical constraints. Stirling engines use regeneration to recycle heat internally. Combined cycle plants use multiple fluids and cascaded cycles to span a more extensive temperature range. Recent research into nano-scale heat engines probes whether thermodynamic efficiencies could be raised in the domain of quantum mechanics.
Regardless of the approach, Carnot’s efficiency is the ultimate goal post – a sometimes taunting reminder of the complex physical limits imposed by thermodynamics and the prize to be reached.
As rising concern over climate change spurs an intense push for cleaner, more efficient energy systems, the Carnot engine’s foundational insights are as relevant as ever to the cutting edge of engineering.
Fast forward to 2024, and UK-based startup Carnot Engines is on a mission to turn Carnot’s theoretical marvel into an absolute powerhouse of the net zero economy.
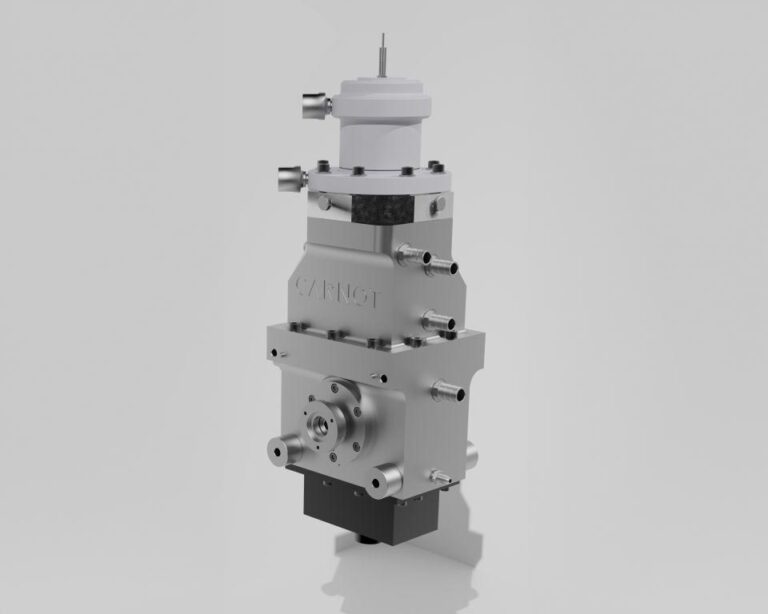
By innovating on materials, design, and manufacturing, they’ve created a heat engine that can achieve 70% of Carnot’s efficiency, nearly doubling the efficiency of conventional engines.
The key is a novel architecture enabling higher temperature differentials and seamless fuel flexibility. “We can run our engines on hydrogen, ammonia, methanol, biofuels – pretty much any fuel,” explains George Hunter, Head of Sales at Carnot.
“In hydrogen mode, the only emission is water vapour. It’s a true zero-emissions solution.”
This fuel-agnostic approach is a significant advantage in a world still hashing out clean fuels’ future. Operators can switch between different fuel options to optimize cost and carbon footprint as technologies and supply chains evolve. It provides a pathway for hard-to-decarbonize sectors like shipping and industry to progress despite uncertainty.
Realizing Carnot’s dream engine has required surmounting major technical hurdles. Advanced ceramics and clever design sidestep the limitations that hindered earlier efforts, like the inability of common engine materials to withstand very high-temperature gradients and pressures.
New manufacturing technologies have also been key. “3D printing allows us to create bespoke parts on demand, and the micro-scale precision of modern machining is essential for minimizing losses,” says Hunter.
Sensors and onboard intelligence fine-tune performance in real time. As Carnot looks to scale, a familiar challenge looms: the skills gap. Amidst a chronic talent shortage, assembling teams with the right expertise in ceramics, advanced manufacturing, power electronics, and more is challenging.
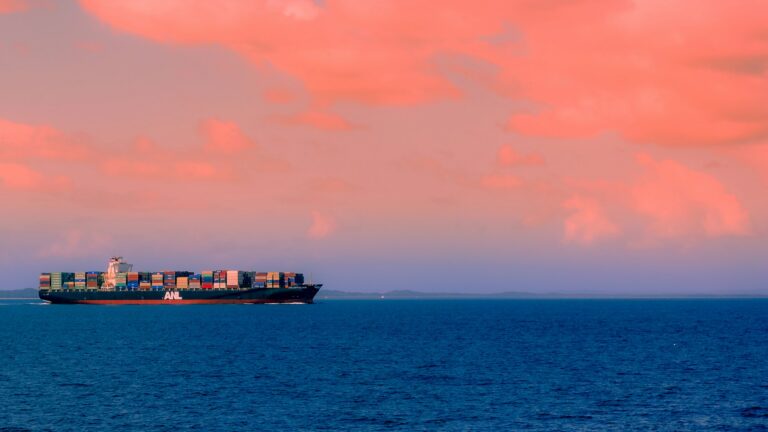
The company is tackling this head-on with a novel apprenticeship program that recruits and trains young technicians. Looking ahead, Carnot engines’ potential applications are exciting. They can replace diesel generators, power long-haul transport, and generate dispatchable clean electricity. Carnot engines offer a cleaner, more efficient alternative anywhere fuel is burned for energy. Their first real-world test is slated for early 2025, with a hydrogen engine powering auxiliary systems aboard a Carisbrooke Shipping vessel.
As Carnot engines come closer to commercial reality, it’s worth considering the long arc of thermodynamic progress they represent. From the coal-fired steam engines that birthed the Industrial Age to the solar thermal plants and fuel cells that may power the next, the pursuit of efficient energy conversion has driven technological progress for centuries.
Carnot’s original vision – an engine that perfectly conserves and converts energy between heat and work – will likely remain just a theoretical ideal, always beyond reach. But in striving toward that ideal, engineers continue to push out the boundaries of the possible, developing ever cleaner and more efficient ways to power human civilization.
This is only becoming more important as the world grapples with the urgent imperative of net zero emissions; Carnot’s 200-year-old idea is needed today in a way it was not when it was conceived. While challenges remain, the reward is huge: an efficient, flexible, zero-emissions successor to the engines that have driven the world for over a century. Amid all the net zero transition turbulence, the elegant cycles that Sadi Carnot sketched long ago still trace a path toward sustainability.
TLDR:
– Carnot heat engines are the most efficient engines allowed by thermodynamics but have been historically impractical to build
– Startup Carnot Engines has made breakthroughs in efficiency and fuel flexibility using advanced materials and manufacturing
– The fuel-agnostic Carnot engine could help decarbonize shipping, power generation and more
– Realizing Carnot efficiency requires overcoming engineering challenges and bridging skills gaps
– As the world pursues net-zero emissions, Carnot’s 200-year-old insights remain powerfully relevant